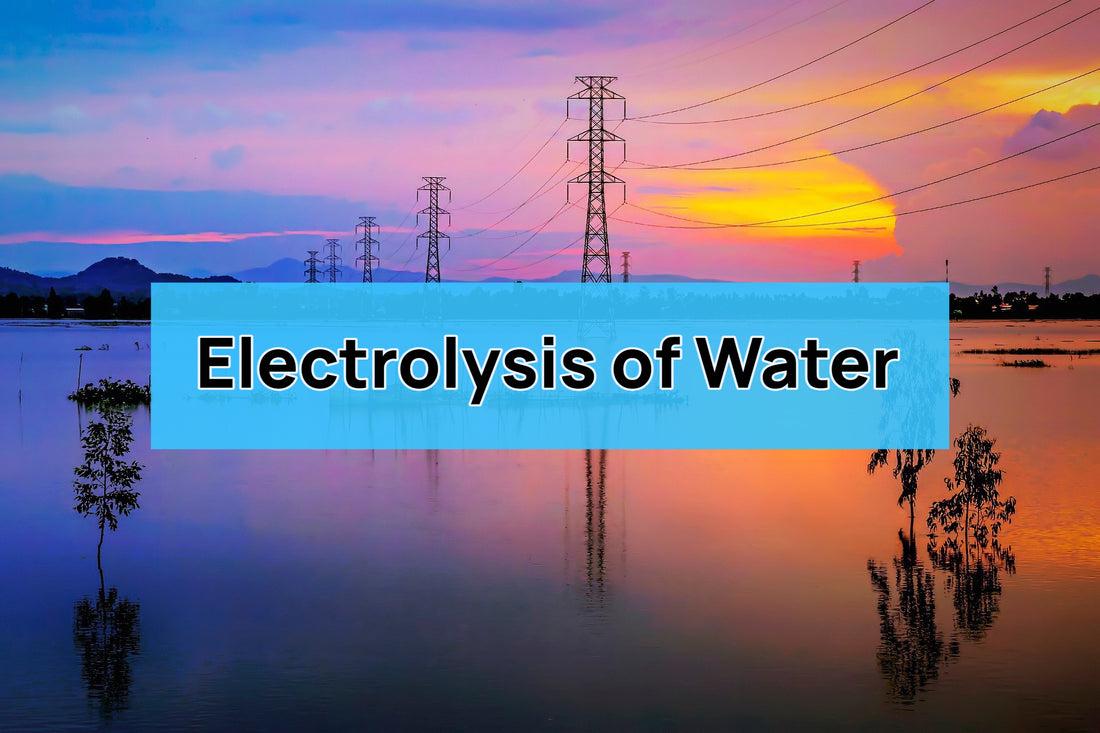
Electrolysis of Water: Understanding the Process
Share
The electrolysis of water is a process that uses an electric current to split water molecules (H₂O) into their constituent elements, hydrogen (H₂) and oxygen (O₂). This is achieved by passing a direct current (DC) through water, typically with an electrolyte added to improve conductivity. The process occurs in an electrolytic cell, where water is broken down at the anode (oxygen is released) and cathode (hydrogen is released). Electrolysis is commonly used for producing hydrogen gas for various industrial applications and research, including hydrogen fuel and hydrogen-rich water for health benefits.
Key Takeaways
- Electrolysis of water offers a carbon-free method for producing hydrogen and oxygen using renewable energy sources.
- Electrolysers come in various sizes, from small-scale units to large-scale facilities, allowing for flexibility in hydrogen production.
- Electrolysis of water offers immense benefits through its use in health and wellness products such as hydrogen water bottles
- Green hydrogen produced through water electrolysis has the potential to revolutionize the energy sector and reduce greenhouse gas emissions.
- Understanding the principles, thermodynamics, and types of electrolysers is crucial for optimizing the efficiency and cost-effectiveness of water electrolysis.
- Water electrolysis holds immense promise for shaping a sustainable future by harnessing renewable energy resources for clean hydrogen production.
Introduction to Electrolysis of Water
Electrolysis of water is a fundamental chemical process that involves using an electrical current to separate water molecules into hydrogen and oxygen gases. This technique is carried out in an electrolytic cell, where water is split into its components—hydrogen at the cathode and oxygen at the anode. The process is widely applied in industries to generate hydrogen for fuel, manufacturing, and even health products like hydrogen-rich water. Electrolysis of water serves as an important method for producing clean energy and exploring sustainable technologies.
Definition of Electrolysis
Electrolysis is a chemical reaction powered by an electric current. In water splitting, it decomposes water into hydrogen and oxygen gases. The reaction can be simplified as follows:
2H2O (l) → 2H2 (g) + O2 (g)
For every two moles of water, two moles of hydrogen and one mole of oxygen are produced. Pure water doesn't conduct electricity well. To improve conductivity, an electrolyte like sulfuric acid or sodium nitrate salt is added.
Importance of Electrolysis in Hydrogen Production
Hydrogen from electrolysis could be a clean, sustainable energy source. If the electricity comes from renewables, the hydrogen is green and emissions-free. This makes electrolysis a key method for achieving the Hydrogen Energy Earthshot goal of reducing clean hydrogen costs to $1 per kilogram in a decade.
Half-Reaction | Electrode | Product |
---|---|---|
Reduction | Cathode | Hydrogen gas (H2) |
Oxidation | Anode | Oxygen gas (O2) |
In alkaline water electrolysis, the cathode produces hydrogen gas and hydroxide ions. The anode releases oxygen gas. The standard potential of a water electrolytic cell is -1.229 V at 25 ˚C and pH = 0. Yet, in practice, higher potentials are needed to overcome various barriers.
As Australia advances in renewable energy, water splitting through electrolysis will be vital for clean hydrogen production and storage. Understanding this process is key to a sustainable, energy-efficient future.
History of Electrolysis of Water
The journey of water electrolysis began in the late 18th century. Scientists started exploring the idea of splitting water molecules with electricity. In 1789, Dutch chemists Jan Rudolph Deiman and Adriaan Paets van Troostwijk achieved the first water electrolysis using an electrostatic generator.
The breakthrough came with the invention of the voltaic pile by Italian physicist Alessandro Volta in 1800 (pictured below). Weeks later, British scientists William Nicholson and Anthony Carlisle successfully used the voltaic pile to electrolyze water. This marked a significant milestone in the history of electrolysis.
In 1806, Humphry Davy reported on extensive experiments with distilled water electrolysis. His findings significantly advanced our understanding of the process. As the 19th century progressed, more scientists and inventors contributed to water electrolysis technology. In 1869, Belgian inventor Zénobe Gramme invented the Gramme machine (pictured below), making electrolysis more affordable for hydrogen production.
Russian physicist Dmitry Lachinov developed an industrial method for synthesizing hydrogen and oxygen through electrolysis in 1888. By the early 20th century, water electrolysis had become a well-established process. By 1902, over 400 industrial units were operational, driven by growing hydrogen demand in industries like ammonia production and oil hydrogenation.
"The discovery of the decomposition of water by the electrical spark is altogether the greatest discovery that has been made in chemistry since the science began."
- Humphry Davy, English chemist (1778-1829)
Advancements in materials science and engineering in the 20th century led to more efficient and cost-effective electrolyzers. Today, water electrolysis remains crucial for green hydrogen production, with alkaline water electrolysis (AWE) being the dominant solution. Research and development efforts aim to enhance efficiency, durability, and scalability. This supports the growing demand for clean energy and the transition to a low-carbon economy.
Year | Event |
---|---|
1789 | First demonstration of water electrolysis by Deiman and Troostwijk |
1800 | Invention of the voltaic pile by Volta |
1800 | Nicholson and Carlisle successfully electrolyze water using the voltaic pile |
1806 | Humphry Davy reports results of distilled water electrolysis experiments |
1869 | Invention of the Gramme machine by Zénobe Gramme |
1888 | Dmitry Lachinov develops an industrial method for hydrogen and oxygen synthesis through electrolysis |
1902 | Over 400 industrial water electrolysis units in operation |
As electrolysis technology evolved through the 19th and 20th centuries, it paved the way for significant advancements in hydrogen production and its various applications. In recent decades, the focus has expanded from industrial-scale hydrogen synthesis to more consumer-friendly innovations, such as hydrogen water bottles, which utilise electrolysis to offer potential health benefits. Below is a timeline highlighting the more contemporary milestones in the history of water electrolysis and its role in modern wellness technology:
Year | Event |
---|---|
1939 | First large-scale hydrogen production plant using electrolysis built in Norway |
1960 | Research on using electrolysis to produce hydrogen for fuel cells in aerospace applications advances |
1990 | Development of PEM (Proton Exchange Membrane) electrolysis technology for more efficient hydrogen production |
2007 | Dr. Shigeo Ohta publishes a study on the health benefits of molecular hydrogen, sparking interest in hydrogen water |
2010 | The first commercial hydrogen water bottles using electrolysis technology are introduced to the market |
2020 | Growing popularity of hydrogen water bottles for their potential health benefits, utilizing advanced SPE/PEM electrolysis technology to produce high-concentration hydrogen water |
2023 | Widespread adoption of hydrogen water bottles as part of wellness routines, with improved efficiency and design, making hydrogen-infused water more accessible to consumers. |
Principles of Electrolysis of Water
The process of water electrolysis decomposes water into hydrogen and oxygen gases using an electric current. It involves two key reactions: the reduction at the cathode and the oxidation at the anode. Exploring these principles is essential to comprehend the electrolysis of water.
Reduction and Oxidation Reactions
At the core of water electrolysis are the reduction and oxidation reactions. The reduction reaction occurs at the cathode, where electrons convert hydrogen cations into hydrogen gas. Conversely, the anode undergoes oxidation, producing oxygen gas and releasing electrons to close the circuit.
The oxidation reaction at the anode is described by:
2H2O(l) → O2(g) + 4H+(aq) + 4e-
Balancing the Half-Reactions
To achieve equilibrium, the reduction and oxidation half-reactions must be paired. This ensures electron balance between the anode and cathode. An electrolyte, such as an acid or base, is crucial for this balance, providing the necessary ions.
The overall equation for water electrolysis is:
2H2O(l) → 2H2(g) + O2(g)
Efficiency in electrolysis depends on several factors, including electrode type, electrolyte composition, and voltage. Ongoing research aims to improve these aspects for better water electrolysis performance.
The following table outlines the key aspects of the reduction and oxidation reactions in water electrolysis:
Reaction | Electrode | Equation |
---|---|---|
Reduction | Cathode | 2H+(aq) + 2e- → H2(g) |
Oxidation | Anode | 2H2O(l) → O2(g) + 4H+(aq) + 4e- |
Understanding the reduction and oxidation reactions highlights the potential of water electrolysis. It showcases a sustainable method for generating clean hydrogen fuel.
Thermodynamics of Electrolysis of Water
The thermodynamics of water electrolysis are key to understanding the energy changes and efficiency of the process. By examining the standard potential, Gibbs free energy, and other thermodynamic parameters, we gain valuable insights into the electrochemical splitting of water molecules.
Standard Potential of the Water Electrolysis Cell
The standard potential of the water electrolysis cell is crucial for understanding the minimum voltage needed for the reaction to start spontaneously. At standard temperature and pressure (STP) conditions of 1 bar and 273.15 K, the standard potential is -1.229 V. This value is derived from the Gibbs free energy change (∆G) during the process, which is 237.24 kJ/mol at 25 °C. The relationship between the standard potential (E°) and Gibbs free energy is given by the equation ∆G° = -nFE°, where n is the number of electrons transferred and F is the Faraday constant (96500 Cmol⁻¹).
It's important to note that the standard potential is a thermodynamic quantity and doesn't account for kinetic factors like activation energy and overpotential. In practice, a higher voltage is needed to overcome these barriers and drive the water electrolysis reaction at a reasonable rate. A deep understanding of the thermodynamics of water electrolysis is essential for optimising the efficiency and performance of electrolysers.
Gibbs Free Energy Calculations
The Gibbs free energy change (∆G) is a critical thermodynamic quantity that determines the spontaneity and direction of the water electrolysis reaction. At standard conditions, the Gibbs free energy change for water electrolysis is positive (∆G° = 237.24 kJ/mol), indicating that the reaction is nonspontaneous and requires an external energy input. The Gibbs free energy is related to the enthalpy change (∆H) and entropy change (∆S) through the equation ∆G = ∆H - T∆S, where T is the absolute temperature.
The enthalpy change (∆H) represents the total energy change during the electrochemical decomposition of water, including the heat of formation of water (∆ f H° g , H 2 O) and the heat of vaporization (∆ vap H°, 25°C). At 25 °C, the enthalpy change for water electrolysis is 285.83 kJ/mol. The entropy change (∆S) reflects the increase in disorder as water molecules are split into hydrogen and oxygen gases, and its value is 163 J/K mol at standard conditions.
Thermodynamic Parameter | Value | Units |
---|---|---|
Gibbs Free Energy Change (∆G°) | 237.24 | kJ/mol |
Enthalpy Change (∆H°) | 285.83 | kJ/mol |
Entropy Change (∆S°) | 163 | J/K mol |
By calculating the Gibbs free energy change at different temperatures and pressures, we can predict the thermodynamic feasibility of water electrolysis under various operating conditions. This information is valuable for selecting the appropriate electrolyser technology and optimizing the process parameters to achieve high efficiency and hydrogen production rates.
Role of Electrolytes in Electrolysis of Water
Electrolytes are vital in water electrolysis, crucial for hydrogen production. We'll explore their role in improving conductivity, discuss common types, and examine solid polymer electrolytes.
Conductivity Enhancement
An aqueous electrolyte significantly boosts water conductivity during electrolysis. It does this by breaking down into cations and anions, enabling electricity flow. This is key for efficient electrolysis operation.
Various cations, like Li+, Rb+, K+, and Mg2+, are suitable due to their lower electrode potential than H+. The right electrolyte choice depends on factors like chemical reactivity, cost, and performance.
Common Electrolytes Used
Strong acids and bases are often used in water electrolysis. Sulfuric acid (H2SO4) is favored for its strong dissociation and conductivity. Potassium hydroxide (KOH) and sodium hydroxide (NaOH) are also common in alkaline electrolyzers.
A study by Nikhilesh Mukherjee highlights the electrolyte's critical role in hydrogen production efficiency and cost. The right choice is essential for sustainable hydrogen production. (Source)
Solid Polymer Electrolytes (SPE) Technology
Solid polymer electrolytes, like Nafion, are gaining attention. They offer advantages over liquid electrolytes. Nafion, a fluoropolymer-copolymer, has excellent proton conductivity and stability.
With a suitable catalyst on each Nafion side, efficient electrolysis occurs at just 1.5 volts. This makes solid polymer electrolytes, especially in PEM electrolyzers, an attractive option.
Electrolyte | Conductivity (S/cm) | Advantages |
---|---|---|
Sulfuric Acid (H2SO4) | 0.8 | High conductivity, strong dissociation |
Potassium Hydroxide (KOH) | 0.6 | Suitable for alkaline electrolysers |
Sodium Hydroxide (NaOH) | 0.5 | Commonly used in alkaline electrolysers |
Nafion | 0.1 | Solid polymer electrolyte, low voltage requirement |
The electrolyte choice is crucial in water electrolysis systems. Understanding their role and exploring options like Nafion can enhance hydrogen production efficiency and performance.
What is SPE/PEM Technology
SPE/PEM technology refers to Solid Polymer Electrolyte (SPE) and Proton Exchange Membrane (PEM), both of which are crucial components in the electrolysis of water. In this process, a solid polymer membrane allows protons (H⁺) to pass through while preventing gases like hydrogen and oxygen from crossing, ensuring efficient hydrogen production. This technology is used in applications such as hydrogen fuel cells and hydrogen water bottles, where it facilitates the clean and efficient generation of hydrogen.
To explore more about how SPE/PEM technology works and its various applications, check out our detailed article on SPE/PEM technology.
Electrolysis of Water: The Process
The electrolysis of water decomposes H2O molecules into hydrogen (H2) and oxygen (O2) gases with an electric current. This occurs in an electrolytic cell, which has two electrodes (cathode and anode) in an electrolyte solution. The solution typically includes water and an ionic compound like an acid, base, salt, or SPE (solid polymer electrolyte) technology to enhance conductivity.

Applying a direct current to the electrodes triggers a redox reaction in the water molecules. At the cathode, positively charged hydrogen ions (H+) gain electrons and are reduced to hydrogen gas (H2). Meanwhile, at the anode, negatively charged hydroxide ions (OH-) lose electrons, oxidizing to oxygen gas (O2) and water (H2O).
The overall reaction for the electrolysis of water is:
2H2O(l) → 2H2(g) + O2(g)
Hydrogen generation occurs at the cathode, while oxygen generation happens at the anode. The amount of hydrogen produced is twice that of oxygen. Both are directly proportional to the electrical charge passed through the electrolyte solution, as per Faraday's laws of electrolysis.
Several factors affect the electrolysis process's efficiency and rate. These include:
- Electrode material and surface area
- Electrolyte composition and concentration
- Applied voltage and current density
- Temperature and pressure
- Presence of catalysts or impurities
Optimising these parameters is essential for maximizing hydrogen and oxygen generation. It also helps in reducing energy consumption and side reactions. Some key statistics related to the electrolysis of water are:
Parameter | Value |
---|---|
Minimum potential difference required | 1.23 volts |
Typical potential difference used | 1.5 volts |
Standard potential of water electrolysis cell (25°C, pH 0) | -1.229 volts |
Temperature of hydrogen gas released | Approximately 2,800°C |
By grasping the principles and optimising the electrolysis process, we can efficiently produce hydrogen and oxygen gases from water. This paves the way for clean energy storage and utilisation in various applications, such as fuel cells and industrial processes.
Types of Electrolysers
In the quest for sustainable hydrogen, electrolyzers are crucial. They split water into hydrogen and oxygen. The main types are alkaline, proton exchange membrane (PEM), and solid oxide electrolysers. These are key to the green hydrogen revolution, with efficiency and scalability crucial for clean energy's future.
Alkaline Electrolysers
Alkaline electrolysers lead in water electrolysis technology. They use potassium hydroxide (KOH) to transport hydroxide ions. Hydrogen is produced at the cathode, and oxygen at the anode. They dominate the market, with nearly two-thirds of global capacity.
Operating at 30 bar, they have an efficiency of 50-60%. This makes them ideal for large-scale hydrogen production.
Proton Exchange Membrane (PEM) Electrolysers
PEM electrolysers are known for their high efficiency and compact design, using a solid plastic material as the electrolyte, which conducts protons but not gases. In this process, water is split at the anode, producing oxygen and hydrogen ions. These ions travel to the cathode, where they form hydrogen gas.
Representing one-fifth of the global capacity, PEM electrolysers achieve an 80-90% efficiency rate. However, their high cost due to the use of platinum and iridium catalysts remains a drawback. This same technology is used in hydrogen water bottles to generate molecular hydrogen for consumption.
Solid Oxide Electrolysers
Solid oxide electrolysers (SOEs) operate at high temperatures, between 500-850°C. They use a ceramic material, like yttria-stabilized zirconia (YSZ), as the electrolyte. At these temperatures, the ceramic becomes conductive to oxygen ions.
Hydrogen is produced at the cathode, and oxygen at the anode. SOEs have shown higher efficiencies than alkaline and PEM electrolysers. Their high operating temperature reduces the energy needed for water splitting. Yet, material stability and system complexity are challenges.
Electrolyser Type | Operating Temperature (°C) | Efficiency Range (%) | Market Share (%) |
---|---|---|---|
Alkaline | 70-90 | 50-60 | 67 |
PEM | 50-80 | 80-90 | 20 |
Solid Oxide | 500-850 | 90-100 | - |
The transition to a greener future hinges on electrolyzer technology choice. Factors like cost, efficiency, scalability, and integration with renewables are key. Research aims to enhance performance and lower costs, making green hydrogen more viable. Advances in alkaline, PEM, and solid oxide electrolysis are crucial for hydrogen's clean energy potential.
Advantages and Disadvantages of Different Electrolysers
Each electrolyser type has unique advantages and disadvantages. Understanding these differences is essential for choosing the right electrolyser for a specific application. This involves considering electrolyser efficiency and renewable energy integration.
Efficiency Comparison
Efficiency is a critical factor in selecting an electrolyser. Here's a comparison of different types' efficiency:
Electrolyser Type | Efficiency | Scale |
---|---|---|
PEM (Proton Exchange Membrane) | High | Small-scale |
Alkaline | Medium | Medium to large-scale |
AEM (Anion Exchange Membrane) | High | Flexible |
SOEC (Solid Oxide Electrolysis Cell) | High | Large-scale |
PEM electrolysers excel in small-scale hydrogen production due to their high efficiency. Alkaline electrolysers, used for over a century, are ideal for medium- to large-scale hydrogen production. AEM electrolysis offers high efficiency and low costs, while SOEC operates at high efficiency and temperature, making it suitable for large-scale production.
Suitability for Renewable Energy Integration
Integrating electrolysers with renewable energy sources is key for sustainable hydrogen production. Different types have varying suitability levels:
- PEM electrolysers are ideal for fluctuating renewable energy sources due to their quick response and immediate start-up.
- Alkaline electrolysers are cost-effective and preferred for small-scale hydrogen production with renewable energy.
- SOEC electrolysis is suitable for hydrogen production in areas with abundant heat, such as industrial sites and nuclear power plants.
- AEM technology combines alkaline and PEM, requiring no rare metals and offering flexibility for various renewable energy sources.
In conclusion, the choice of electrolyzer depends on application-specific requirements. Factors like efficiency, scale, and compatibility with renewable energy sources are crucial. By carefully considering these, we can optimise hydrogen production and contribute to a sustainable future.
Applications of Electrolysis of Water
The electrolysis of water has a broad range of applications, spanning from industrial hydrogen production to personal health and wellness. By using electricity to split water into its component elements—hydrogen and oxygen—electrolysis plays a crucial role in numerous fields. The versatility and clean nature of the process make it essential for industries and technologies aiming to reduce environmental impacts and create more efficient energy solutions.
Hydrogen Fuel Production
One of the most significant applications of water electrolysis is the production of hydrogen fuel. Green hydrogen, produced via electrolysis powered by renewable energy sources, is gaining attention as a clean alternative to fossil fuels. In this process, the electrolysis of water creates hydrogen gas, which can be stored and used in fuel cells for transportation, energy storage, and industrial processes. This method is critical in the global effort to reduce carbon emissions, as hydrogen-powered vehicles and hydrogen fuel cells offer zero-emission alternatives to conventional energy systems.
Industrial Oxygen Supply
Electrolysis also serves as a method of producing high-purity oxygen. The oxygen generated during electrolysis can be captured and used in various industries, including medical oxygen supply, metal processing, and chemical manufacturing. Industrial-scale water electrolysers are often employed to meet the demand for oxygen in sectors that require large volumes of high-purity gases for combustion processes and chemical reactions.
Hydrogen Water Bottles
A more recent and personal application of electrolysis is in hydrogen water bottles, which have gained popularity in the wellness industry. These bottles use a scaled-down version of Proton Exchange Membrane (PEM) electrolysis to infuse water with molecular hydrogen. The PEM technology allows hydrogen ions to pass through the membrane, where they combine with electrons to form hydrogen gas (H₂), which dissolves into the water.
Hydrogen water bottles are believed to offer various health benefits, including reducing oxidative stress and promoting recovery after physical exertion. While ongoing research continues to explore these potential hydrogen water benefits, hydrogen water bottles are increasingly being adopted by consumers looking for wellness solutions. The convenience and portability of these bottles make them a popular choice for health-conscious individuals.
Water Purification
Electrolysis can also be used in water purification systems. The electrolysis process can generate chlorine, which acts as a disinfectant, helping to kill harmful bacteria and pathogens in water. This method is commonly used in swimming pools, drinking water systems, and wastewater treatment plants to ensure that water is safe for human consumption and use. Electrolysis-based water purification systems offer an environmentally friendly alternative to chemical-based methods, reducing the need for hazardous chemicals.
Future Prospects for Electrolysis of Water Applications
As technologies advance and the world increasingly focuses on sustainability, the applications of water electrolysis are expected to expand even further. From large-scale hydrogen production to small-scale devices like hydrogen water bottles, electrolysis holds the potential to revolutionise energy, healthcare, and environmental solutions. With ongoing research into more cost-effective materials and methods, the future of electrolysis looks bright in both industrial and personal applications.
Current Research and Future Prospects
The field of water electrolysis is rapidly advancing, with a focus on overcoming cost and efficiency challenges. Researchers aim to meet the Hydrogen Shot's ambitious goal of $1/kg H2 by 2030. This requires a concerted effort in research and development.
Overcoming Challenges in Cost and Efficiency
Reducing electrolyser capital costs and improving energy efficiency are key research areas. Understanding the performance, cost, and durability trade-offs is crucial. We aim to develop innovative solutions that optimise the process.
Efforts focus on identifying and addressing high-cost factors. This includes developing low-cost, high-performance electrode materials and optimising catalyst loading and distribution. Enhancing membrane stability and improving stack design are also priorities.
- Developing low-cost, high-performance electrode materials
- Optimizing catalyst loading and distribution
- Enhancing membrane stability and conductivity
- Improving stack design and manufacturing processes
Increasing electrolyser energy efficiency is vital for green hydrogen adoption. Researchers are exploring new catalyst materials and advanced membrane technologies. They also aim to minimise energy losses and improve overall efficiency.
Synergy with Renewable Energy Power Generation
Hydrogen fuel and electric power generation at renewable energy sites offer a sustainable future. This synergy optimises clean energy resource utilisation and reduces fossil fuel reliance.
Research focuses on developing efficient hydrogen production systems for renewable energy sources. Advanced control strategies and energy storage solutions are being explored. This ensures a stable green hydrogen supply, even during low renewable energy periods.
The synergy between water electrolysis and renewable energy power generation is a game-changer for the hydrogen economy. It allows us to harness the full potential of clean energy resources while reducing the carbon footprint of hydrogen production.
Co-locating hydrogen production facilities with renewable energy sites minimizes transmission losses. This approach reduces hydrogen production costs and aids in decarbonising sectors like transportation and industry.
Parameter | Value |
---|---|
Electricity consumption per kg H2 | 55 kWh |
Lower calorific value of H2 | 33 kWh/kg |
PEM electrolyser stack temperature (ISS) | 335 K |
PEM electrolyser cell voltage (ISS) | 1.72 V |
Oxygen production rate (ISS) | 2.3-9.2 kg/day |
Advancing water electrolysis and its synergy with renewable energy is promising. Through dedicated research and collaborative efforts, we're creating a sustainable energy future. This future is bright, with green hydrogen production leading the way in Australia and beyond.
Final Thoughts on Electrolysis of Water
Reflecting on water electrolysis and its role in sustainable hydrogen production, we see its immense potential for clean energy. This technology splits water into hydrogen and oxygen using electricity. It's a promising method for renewable energy storage. Yet, to fully benefit from it, we must tackle the issues of cost and efficiency.
Researchers are working on improving electrolyzers' performance and reducing costs. They focus on alkaline, proton exchange membrane (PEM), and solid oxide electrolyzers. Each has its strengths and weaknesses. The goal is to enhance their efficiency and compatibility with renewable energy systems. The global electrolyzer capacity is expected to soar, with 35 GW by the mid-2020s and 134 GW by 2030, as stated in the Global Hydrogen Review 2022 by the International Energy Agency.
To maximize water electrolysis for sustainable hydrogen, pairing it with renewable energy is crucial. Using solar, wind, or hydropower for clean electricity ensures green hydrogen production. This approach helps reduce greenhouse gas emissions. As we invest in research, combining electrolysis with renewable energy will be essential for a sustainable energy future.
In summary, water electrolysis is a crucial step towards clean, sustainable energy. Despite challenges, advancements in electrolyzer technology and integration with renewables are encouraging. As we address cost and efficiency hurdles, sustainable hydrogen production through electrolysis will be vital for our transition to a low-carbon economy.
Frequently Asked Questions on the Electrolysis of Water
What is electrolysis of water?
Electrolysis of water involves using electricity to split water into hydrogen and oxygen gases. It's a method for creating carbon-free hydrogen from renewable and nuclear energy.
How does electrolysis of water work?
Water electrolysis uses an electric current to split water. At the cathode, electrons reduce hydrogen cations to form hydrogen gas. Meanwhile, at the anode, an oxidation reaction produces oxygen gas and completes the circuit.
What are the main types of electrolyzers?
There are three main types: alkaline, proton exchange membrane (PEM), and solid oxide electrolyzers. Each operates with a different electrolyte and under distinct conditions.
What role do electrolytes play in water electrolysis?
Electrolytes improve water conductivity in electrolysis by breaking down into cations and anions. This allows for continuous electricity flow. Common electrolytes include strong acids and bases like sulfuric acid and potassium hydroxide.
What are the advantages of using electrolysis for hydrogen production?
Hydrogen from electrolysis can have zero greenhouse gas emissions, depending on the electricity source. It also aligns with dynamic, intermittent power from renewables like wind.
What challenges does water electrolysis face in terms of cost and efficiency?
Current research aims to meet the Hydrogen Shot's $1/kg H2 target by 2030. This involves understanding performance, cost, and durability trade-offs. Efforts focus on reducing costs, enhancing efficiency, and extending operational life.
What is the future potential of electrolysis in sustainable hydrogen production?
As research progresses and costs fall, electrolysis could be key in the clean energy transition. More efficient, cost-effective electrolyzers and renewable energy growth are essential for its widespread adoption.
Disclaimer
The information provided in this blog is for educational and informational purposes only and is not intended as medical advice. Please consult with a healthcare professional before making any changes to your diet, lifestyle, or health regimen. Results from using hydrogen water may vary, and individual experiences may differ.